Status of Power System Transformation 2019
Power system flexibility
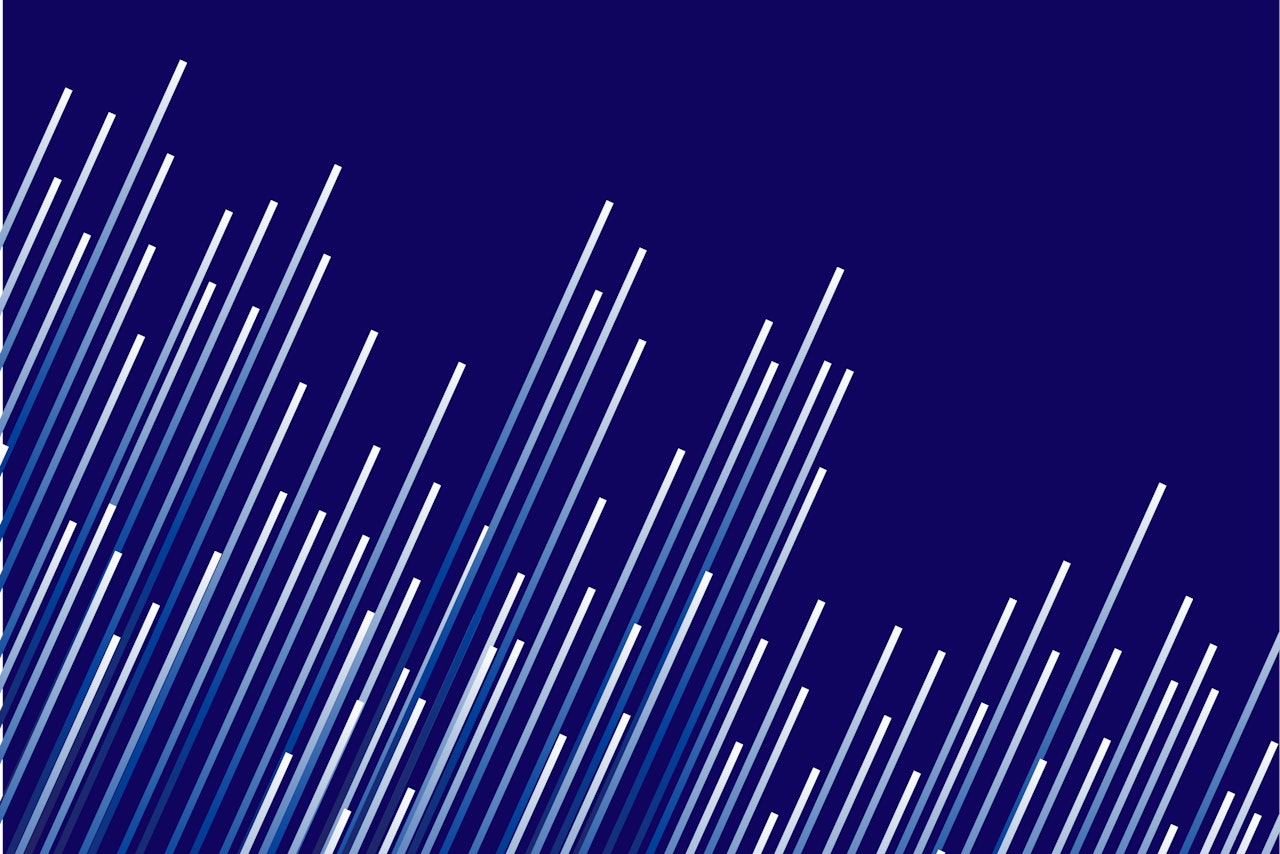
About this report
Importantly, all power system assets, including variable renewable energy, can provide flexibility services, if enabled by proper policy, market and regulatory frameworks. These assets include power plants, electricity networks, energy storage and distributed energy resources. A wealth of known strategies, approaches and instruments can be readily applied and adapted to power systems. These include modifications to: energy strategies; legal frameworks; policies and programmes; regulatory frameworks; market rules; system operation protocols; and connection codes. Moving forward, updating system flexibility policies to match the pace of technological development can help to accelerate global PST, while ensuring that all classes of power system assets are able to receive fair remuneration for the flexibility services they are capable of providing.
Executive summary
Power systems around the world are undergoing significant change, driven particularly by the increasing availability of low-cost variable renewable energy (VRE), the deployment of distributed energy resources (DER), advances in digitalisation and growing opportunities for electrification. These changes can be managed in the process of power system transformation (PST).
Status of Power System Transformation 2019 identifies challenges and opportunities to unlock system flexibility and accelerate PST. A wealth of known strategies, approaches and instruments to support power system flexibility can be readily applied and adapted to power systems.
There are several categories of power system assets that can be utilised to provide flexibility. Conventional power plants are currently the predominant source of system flexibility in modern power systems, and many strategies are available to further “flexibilise” these resources. VRE sources, such as wind and solar photovoltaic power plants, are emerging as a flexibility resource, with several countries recently introducing market reforms and regulations that activate VRE flexibility. Electricity networks remain a critical enabler of system flexibility, and a range of policy and regulatory instruments are available to de-risk new network investments and promote more “system-friendly” deployment of VRE. Energy storage, particularly battery energy storage systems (BESS), are becoming a cost-competitive flexibility provider. Modifications to policy, market and regulatory frameworks ensure BESS can participate in the power system to provide flexibility services. DER offer significant flexibility potential, but may require market and regulatory reforms to provide valuable system flexibility services.
There is an established and quickly growing body of knowledge on the successful management of modern power systems in transition. There is a substantial amount of knowledge, experience and expertise that has been accrued on PST over the past decade. A wealth of measures to support power system flexibility can be readily applied and adapted to power systems, these include modifications to: energy strategies; legal frameworks; policies and programmes; regulatory frameworks; market rules; system operation protocols; and connection codes.
All power system assets can provide flexibility services if enabled by proper policy, market and regulatory frameworks. Even VRE resources are emerging as a flexibility resource. Several countries (e.g. Australia, Ireland, Spain and the United States) have introduced market reforms and regulations that activate VRE flexibility. In the United States, innovative flexibility retrofit investments have been demonstrated in existing conventional power plants, such as hybridisation with BESS. Moving forward, efforts to modify connection codes and market rules will be key for all assets – including power plants, electricity networks, DER and energy storage – to receive fair remuneration for their flexibility services.
Electricity networks remain a critical enabler of system flexibility. Various policy and regulatory instruments can de-risk new network investments in support of system flexibility, and promote more “system-friendly” deployment of VRE. In the United Kingdom, a “cap-and-floor” regulatory regime mitigates investment risk in merchant interconnection projects by protecting investor in years with low market revenue in exchange for capping revenues in high revenue years. Recent analysis of the Chinese power system in 2035 by the International Energy Agency (IEA) shows that increasing co-ordination to make better use of existing regional interconnections could yield significant economic and environmental benefits. In India, a solar parks strategy helps to mitigate grid connection risks for developers while fostering a more system-friendly deployment of solar photovoltaics (PV).
Battery energy storage systems are becoming a cost-competitive flexibility provider. Modifications to policy, market and regulatory frameworks can accelerate BESS deployment and ensure these resources can participate in the power system to provide flexibility services. Further regulatory innovations can help to unlock the multiple value streams of energy storage. Australia, the United Kingdom, the United States and the European Union’s Electricity Directives offer useful examples of regulatory innovations.
Distributed energy resources offer significant flexibility potential but may require market and regulatory reforms. Proactive policy making and innovative regulations can be introduced to enable DER aggregators and streamline enrolment of DER to provide valuable flexibility services. Innovative approaches to enable DER aggregation are emerging, as demonstrated with virtual power plants in Italy and Finland. In Germany, a market-based approach to utilise DER to provide localised flexibility services is being tested to relieve network congestion. In Singapore, the utilisation of DER to provide flexibility services has been enabled through retail market liberalisation.
Introduction
Power systems around the world are undergoing significant change, driven particularly by the increasing availability of variable renewable energy (VRE), the deployment of distributed energy resources (DER), advances in digitalisation and growing opportunities for electrification. These changes can be managed in the process of power system transformation (PST). PST describes the processes that facilitate and manage changes in the power sector in response to these novel trends. It is a process of creating policy, market and regulatory environments, and establishing operational and planning practices that accelerate investment, innovation and the use of smart, efficient, resilient and environmentally sound technology options. PST is often a complex task for policy makers.
This report summarises the findings of the Power System Flexibility (PSF) campaign launched at the 9th Clean Energy Ministerial (CEM9) in Copenhagen in May 2018. The campaign seeks to build momentum among industry and governments to enhance power system flexibility. This report identifies challenges and opportunities to unlock system flexibility and accelerate PST, and provides an overview of the policy, regulatory and market instruments which can be implemented in different power sector contexts to mitigate these challenges.
Power system flexibility has become a global priority
Power system flexibility is defined as “the ability of a power system to reliably and cost-effectively manage the variability and uncertainty of demand and supply across all relevant timescales, from ensuring instantaneous stability of the power system to supporting long-term security of supply” 1,2. Flexibility is already an important characteristic of all power systems. A range of operational, policy and investment-based interventions are available to render modern systems more flexible, thereby facilitating cleaner, and more reliable, more resilient and more affordable energy. However, it is apparent that a greater focus on supporting power system flexibility is needed during PST, in order to promote and facilitate the transition to more affordable, clean, reliable and resilient power systems.
Variable renewable energy is a key driver of system flexibility requirements
The increasing prominence of VRE – and its associated “system integration” issues – is among the most important drivers of PST globally, and different levels of VRE penetration require an evolving approach to providing power system flexibility. The IEA has developed a phase categorisation to capture the evolving impacts that VRE may have on power systems, as well as related integration issues. The integration of VRE can be categorised into six different phases 3. This framework can be used to prioritise different measures to support system flexibility, identify relevant challenges and implement appropriate measures to support the system integration of VRE (Figure 1).
A distinct set of system integration issues are experienced as VRE penetrations increase in power systems
Figure 1. Key characteristics and challenges in the different phases of system integration
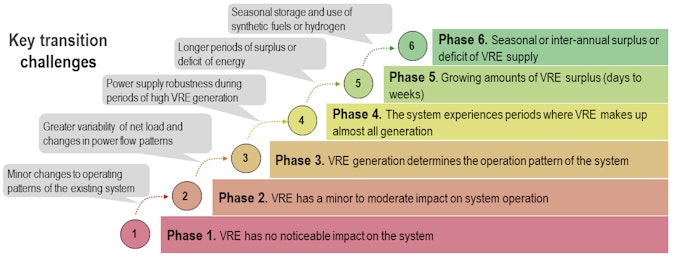
Source: Adapted from IEA (2018a), World Energy Outlook 2018.
Figure 2 presents annual VRE shares and corresponding system integration phases for select countries and regions. Presently, Phase 4 is the highest VRE integration phase that has been achieved in practice. A small number of countries and regions (e.g. Denmark, Ireland and South Australia) have reached Phase 4, but many other power systems are still in Phases 1 and 2 and have 5-10% shares of VRE in annual electricity production. However, the general direction of this transition is already clear: higher phases of system integration are forthcoming for most countries, and reflected in the increased levels of VRE deployment and new national efforts to boost power system flexibility.
VRE is increasingly influencing power system operations around the world
Figure 2. Annual VRE share and corresponding system integration phase in selected countries/regions, 2018
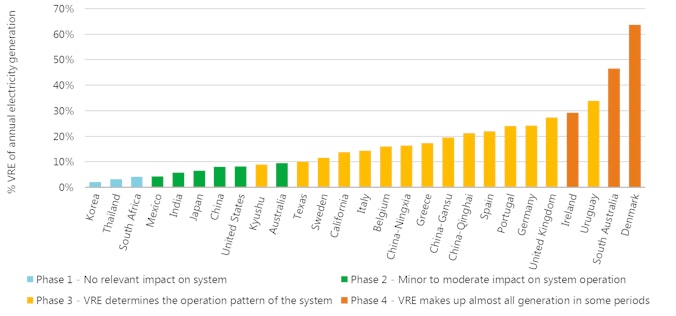
Source: IEA (forthcoming), Renewables 2019: Analysis and Forecasts to 2024. Notes: China = The People’s Republic of China.
VRE is increasingly influencing power system operations around the world.
The share of VRE in many countries has grown over the past few years. In 2015, there were just over 30 countries with an annual generation share of VRE greater than 5%; by 2018, this number had risen to nearly 50 countries. Shares of VRE in many countries and/or regions are expected to rise from 5-10% to 10-20% over the next five years; jurisdictions with shares of 20-40% are also expected to increase significantly as shown in Figure 3 4.
As the number of countries with medium-to-high shares of VRE rises significantly, it is expected that power system flexibility will become a more prominent issue in coming years
Figure 3. Number of countries within annual VRE penetration range, historical and projected
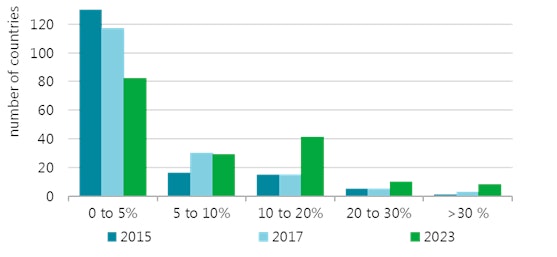
Source: Adapted from IEA (2018b), Renewables 2018: Analysis and Forecasts to 2023.
Key findings
1. Management of power systems in transition
There is an established and quickly growing body of knowledge on the successful management of modern power systems in transition
A substantial amount of knowledge, experience and expertise has been accrued on PST over the past decade. Much of this knowledge has been documented in global reports, including in earlier 21CPP and IEA reports 5; 6. Many of the previously stated core messages to policy makers about supporting system flexibility still apply, including the importance of:
- Mitigating power system flexibility requirements through improved system operations and expanding the geographic footprint of power systems.
- Co-ordinating and integrating planning exercises across power market segments and even economic sectors such as transportation and industry.
- Developing rules for the evolution of power markets that enable and reward system flexibility.
- Leading public engagement, particularly for new transmission projects with long lead-times.
A wealth of strategies, approaches and instruments can be readily applied and adapted to power systems
There are a variety of options for policy, market and regulatory instruments that can boost system flexibility – these options can be grouped into several categories of interventions for policy makers to consider and are depicted in the blue boxes in Figure 4.
The range of measures that are available at different levels play unique, and often complementary roles, in enhancing system flexibility. Ultimately, the institutional context will impact the set of instruments available to support power system flexibility. These measures can be categorised as follows:
Energy strategies are increasingly considering power system flexibility in a more detailed manner to ensure that the flexibility requirements of future power systems are met. For example, the People’s Republic of China (“China”) has targeted nearly 220 GW of thermal power plants for flexibility retrofits and/or performance improvements in its 13th Five-Year Plan (2016-2020) for the power sector.
Legal frameworks provide guidance on roles and responsibilities in the power sector, including the extent of unbundling, competition and privatisation, legal definitions of allowed participants, asset types, and applicable taxes and subsidies. Legal frameworks can also provide high-level objectives and guidance to policy makers, and/or stipulate specific targets or goals. Overarching legal frameworks often require modification to enable participation from aggregated DER and/or energy storage resources. For instance, Chile is currently considering legislation to introduce flexibility in existing and new system assets and to enable the participation of DER in system services.
Policies and programmes are commonly created to support the achievement of specific objectives in established energy strategies, for example the “flexibilisation" of power plant fleets through incentive programmes and/or policy mandates. Policy makers may also fund specific programmes to test or pilot certain innovative approaches to better utilise storage resources or flexibilise demand; one example of this is Italy’s feasibility study on ‘Virtual Storage System’ (discussed later).
Regulatory frameworks and decisions allocate the costs and risk of various utility and private sector undertakings to operate and modernise the power sector. For example, in single buyer markets, standard conditions of power purchase agreements are typically specified by regulations. These frameworks also help to implement specific legal requirements and infrastructure development targets during utility resource planning exercises. An example of this is the Colorado Public Utilities Commission’s adoption of an order requiring utilities to consider energy storage resources in their resource planning and procurement process. Regulatory decisions can also help spur innovation and experimentation for system flexibility. For example, the Spanish regulator issued a decision requiring generators to carry out a series of analyses that characterised the ability of the Spanish wind turbine fleet to provide ancillary services. This first step, motivated by the regulator, ultimately led to the broader participation of wind turbines in the Spanish ancillary services market.
Power sector planning exercises help steer investments in the power system, while also promoting affordability and reliability. Increasingly, power system planning exercises are incorporating assessments of flexibility requirements and integrating across power market segments (e.g. considering both generation and transmission investment together) and economic sectors (e.g. distribution network and transportation plans to deploy charging infrastructure). These integrated approaches can help to uncover smart solutions to reduce flexibility requirements, but policy makers may need to intervene to encourage these kinds of approaches. For example, Thailand’s Ministry of Energy has recently begun to consider system flexibility requirements in the formulation of its 2018 Power Development Plan, assigning the national electric utility to conduct studies on ‘Grid Modernization’, which subsequently led to the launch of flexibility pilot projects.
Retail electricity pricing determines how customers are charged for the electricity they consume and compensated for any electricity injected back into the grid. Changes to retail electricity pricing can remove disincentives for customer-sited DER to participate in aggregation schemes that may otherwise increase their electricity bills. If robustly designed, new retail tariff structures can support system flexibility, while simultaneously increasing revenue to DER owners. For example, Singapore’s Open Electricity Market enables participation in demand response programmes by allowing consumers to choose their retailer, or buy directly from the wholesale market at half-hourly prices 7.
Power market rules and codes 8 determine who can participate in wholesale 9 , ancillary services, and/or capacity markets. They also define a set of common rules for the electricity trade, including how to incorporate technical restrictions on the formation of prices. The modification of energy and ancillary service market price formation methodologies are used to better reward flexibility and are a key strategy for liberalised power systems in transition. The development of a suite of market products to properly reward flexibility in transforming power systems is an ongoing challenge, although existing markets offer a range of possibilities for consideration. In the majority of cases, a key underlying principle is the opening of power markets to all technologies. A number of innovative approaches offer the potential to reward flexibility: the United Kingdom’s recent reform of price settlement rules for electricity imbalances is an example of price signals being improved to better reward flexible assets 10.
System operation protocols specify both the procedures and rules on how a power system is operated. Modifications to operational protocols are a common point of intervention to support power system flexibility and include measures, such as: (a) faster power system operation; (b) increased communication and co-ordination between neighbouring power systems; and (c) utilisation of centralised VRE forecasting systems. For example, in Denmark, the deployment of smart meters and the establishment of a centralised DataHub to facilitate all system transactions have improved the ability of retailers to forecast load and VRE, and simplified the settlement process for DER services.
Connection codes specify the various technical requirements for connecting power system resources and loads to the distribution and transmission infrastructure during normal and exceptional operating conditions. Modifications to connection codes can help ensure that all power system resources are able to connect to the grid and provide flexibility services. For instance, changes to connection codes which require VRE to contribute short-term flexibility services (e.g. primary frequency response) are becoming increasingly common.
A range of approaches and interventions to enhance power system flexibility are available at different levels of decision making
Figure 4. Layers of power system flexibility
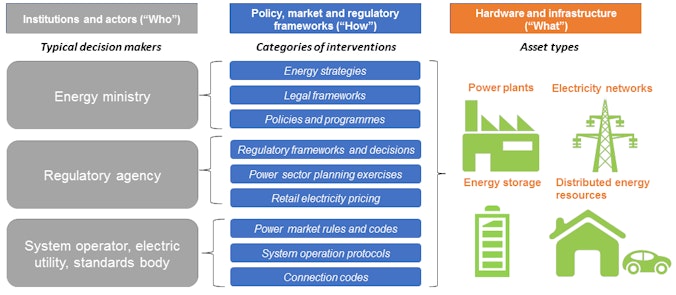
Source: Adapted from (IEA and 21CPP, 2018), Status of Power System Transformation 2018: Advanced Power Plant Flexibility.
Building upon a growing experience base, it is increasingly important to assess options in an integrated manner
As flexibility becomes a more prominent feature of policy dialogues, it is important to approach decision making in a more holistic and integrated manner. There are two key dimensions here. First, by integrating planning across power market segments (e.g. by considering generation and transmission planning expansion decisions in a single exercise), flexibility-related issues can be more holistically evaluated and addressed. Second, as electricity is increasingly used in transport, heating and industry, there is a significant opportunity to “flexibilise” these new sources of electricity demand if transportation planning, building regulations, and other policies and plans are integrated with power sector planning. For example, in 2018, the Australian Energy Market Operator (AEMO) released its first Integrated System Plan (ISP); this ISP for the national transmission network takes into account the generation and growth in distributed PV, electric vehicles, as well as both distributed and utility-scale battery storage 11. Making decisions in isolation may lead to foregone opportunities to support system flexibility, and/or other unintended consequences (e.g. increased network congestion due to unmanaged electric vehicle charging).
Updating system flexibility policies to match the pace of technological development can accelerate global PST
Energy policy and regulation often lag behind technological innovation, which compels forms of “institutional innovation” to play catch up 12. This observation is often relevant in the context of power system flexibility. Policies for increased system flexibility have been introduced in many countries to support PST, and it is possible for policy makers to proactively reform their institutional framework based on the expected or desired development of the power system. For example, in order to facilitate the realisation of its long-term VRE and transport electrification targets, Chile is currently considering the introduction of two bills: the first bill (the Flexibility Bill) has two objectives, namely setting the right incentives for flexible behaviour of existing resources and providing long-term signals for investment in flexible resources; the second focuses on defining the role of distributed system resources and setting the legal basis for more diverse roles in the provision of system services. These examples show that policy makers can make use of long-term targets and planning exercises to anticipate future system needs and accelerate PST.
2. Enablers of power system flexibility
All power system assets, including VRE, can provide flexibility services if properly enabled by policy, market and regulatory frameworks
There are four key categories of infrastructure assets that provide system flexibility, and include: (a) power plants (both conventional and VRE); (b) electricity networks; (c) energy storage; and (d) DER. Conventional power plants, electricity networks and pumped storage hydropower (PSH) have historically been the primary sources of flexibility. However, the operational protocol improvements that have occurred in VRE power plants, electricity networks and the advent of affordable DER and battery energy storage systems (BESS), are enabling a wider set of flexibility options for consideration. As power systems transition toward higher phases of system integration, these flexibility resources can work together in concert to enhance system flexibility in a cost-effective, reliable and environmental sound manner.13 Achieving this goal typically requires changes to policy, market and regulatory frameworks.
Conventional power plants play a critical role in enhancing system flexibility
Conventional power plants are currently the predominant source of system flexibility being used to accommodate supply and demand variability and uncertainty in modern power systems. Flexible power plant operation can take many forms, from rapidly changing plant output, to starting and stopping more quickly, to turning plant output down to lower levels without triggering a shutdown. There are a diverse range of strategies that can make existing conventional power plants more flexible. These strategies can be categorised into two areas:
- Changes to operational practices for existing plants. Significant new capital investments are not necessarily required to operate power plants more flexibly. Changes to certain plant operational practices – often enabled by improved data collection and real-time monitoring – can be used to unlock latent flexibility in existing plants.
- Flexibility retrofit investments for existing plants. A range of retrofit options are available to improve the various flexibility parameters of power plants. For instance, the strategic coupling of BESS with existing plants is increasingly becoming a viable means of boosting flexibility, both in technical and economic terms.
A key example of an innovative flexibility retrofit investment of an existing power plant is Southern California Edison’s Center Peaker plant in Norwalk, California. In this case, a natural gas peaking power plant was coupled to a 10 MW/ 4.3 MWh battery, enabling the plant to offer spinning reserves without burning any fuel, while also offering valuable frequency response services. The BESS component of the hybridised power plant covers the spinning reserve requirements during the first few minutes required for the gas plant to start-up, after which the plant can ramp up to full capacity while the battery output decreases.
With the right policy, market and regulatory conditions in place, VRE can provide valuable system flexibility services
VRE is often perceived as the key driver of new flexibility requirements. VRE power plants can also provide flexibility services to address a range of operational issues related to power systems; however, this requires adequate technical requirements, and in some cases economic incentives, to enable the utilisation of their full range of technical capabilities.
First, it is necessary to have appropriate connection codes that specifically require VRE to provide flexibility services. Due to their highly technical nature, connection codes rarely receive adequate policy attention – amending them can help to increase the visibility and controllability of VRE resources to system operators 14; 15. Second, given that VRE resources are commonly remunerated on a volumetric basis for the energy they produce, and may in some cases provide flexibility services, which in turn require reductions in energy production, it may be necessary to ensure that VRE generators are remunerated fairly for providing flexibility services, just as conventional power plants are.16
Generally, advanced VRE resources can be operated flexibly by either running at full output and dispatching downward when needed (“downward dispatch”), or running at a reduced output and using this “headroom” to be dispatched upward or downward when needed (“full flexibility”). This has been tested and studied in a number of jurisdictions such as California, Chile and Puerto Rico 17; 18. A recent study by E3examines the impact of these operating modes has been studied in the Tampa Electric Company (TECO) system in Florida 19 (Figure 5). The study shows that flexible operation of solar PV resources provides greater operational cost savings as annual solar PV penetration increases on the grid, and also that cost savings are more significant when solar PV power plants are operated in “full flexibility” mode.
Implementing more flexible VRE generators can provide significant cost savings to the power system, particularly as VRE penetration increases
Figure 5. Modelled annual operational cost savings for the TECO power system in Florida, as a percentage of total system operational costs without solar PV generation
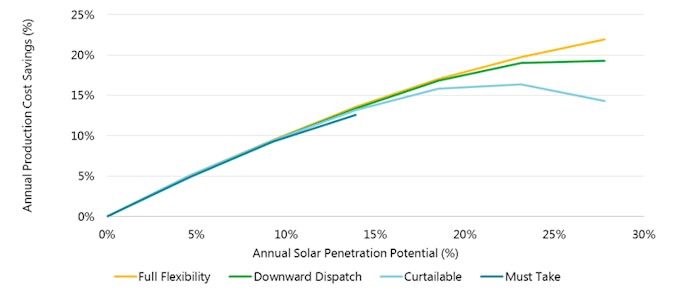
Source: Energy and Environmental Economics, Inc. (2018), Investigating the Economic Value of Flexible Solar Power Plant Operation.
An increasing number of countries are introducing market reforms and regulations that activate flexibility from VRE resources. For instance, a 2018 order from the U.S. Federal Energy Regulatory Commission (FERC) now requires wind and solar resources to provide primary frequency response services. Under the National Electricity Rules in Australia, connection codes require grid-connected VRE plants to provide frequency control ancillary services (FCAS) within each 5 minute dispatch period, which has helped to unlock the technical potential of VRE plants. Spain offers an additional example of enrolling wind generation in flexibility services. For example, Spanish utility Endesa makes use of the geographic diversity of its wind fleet through a virtual power plant approach 20. Solutions and approaches such as these are helping to unlock VRE’s flexibility potential in power systems worldwide.
Market design can evolve to better value the flexible capabilities of power plants
The role of power plants in many power systems is transitioning towards more flexible modes of operation and, at times, reduced operating hours. While existing power plants may offer increasingly important flexibility services to the power system, reductions in energy sales are leading to concerns on their financial viability, calling for the need to re-evaluate how they are rewarded in many markets. This is particularly the case for power systems with wholesale markets; in many of these settings, there are ongoing debates about access to revenue sources to explicitly reward flexibility. A number of options exist to reward flexibility: for example, the current energy market reform of Ontario independent electricity system operator (IESO) seeks to better match flexibility requirements with existing system resources through the introduction of a “Day-Ahead Market” and “Enhanced Real-Time Unit Commitment” to complement its real-time market. More comprehensive, but potentially more debatable measures, include the introduction of scarcity pricing – as adopted in the Electricity Reliability Council of Texas (ERCOT) – or capacity-based remuneration mechanisms. In either case, policy makers can ensure that new revenue sources reward power plants capable of meeting a system’s most critical flexibility requirements rather than just meeting the system peak.
3. Electricity networks
Electricity networks remain a critical enabler of system flexibility
The benefits of electricity networks and interconnections (intra- and inter-regional) cut across all aspects of the power sector, including: (a) improved security of supply; (b) improved system efficiency; and (c) improved integration of VRE resources 21; 22. Electricity networks enable system flexibility by allowing a broader set of flexible hardware resources to be shared across different geographical regions. For example, interconnection allows for an increase in demand to be met by a generator in a neighbouring region during periods when local generation resources are already at maximum output. In addition, electricity networks allow other flexibility options to be shared across a wider area, such as a large energy storage facility that stores excess VRE generation from a power plant located hundreds of kilometres away. Interconnections play an important role in providing flexibility as power systems transition toward higher VRE integration phases. VRE resources typically have a smoother aggregate profile that is easier to integrate across a larger region. Today, significant flexibility resources are still being underutilised due to transmission and interconnection bottlenecks. Leaders have a critical role to play in supporting grid interconnectivity which enables power system flexibility.
Policy and regulatory instruments can help to de-risk transmission investments and unlock flexibility
A typical transmission infrastructure project takes about 10 years23 to develop and build, and calls for a significant amount of research and numerous studies before permits are granted by regulators. Given the long timeframe for project development, it is important for policy makers to proactively lead public engagement and promote a sense of confidence that projects will ultimately be developed, allowing developers to launch feasibility studies and formulate initial business cases. In this context, policies such as the non-binding EU target of 15% interconnection can provide a useful signal to support investor confidence in projects. In addition, the European Union regional power system development plan, the Ten-Year Network Development Plan (TYNDP) assesses the benefits of increased interconnection across the European Union and can help support the case for interconnection projects.
In some cases, transmission projects designed to support power system flexibility may require regulatory intervention to mitigate some investment risks and activate project development. For instance, the Greenlink Interconnector project is a proposed 500 MW subsea interconnector between the United Kingdom and Ireland and is planned to be operational in 2023 24. Greenlink is intended to balance VRE resources and enable efficient use of flexibility resources across the two systems. The United Kingdom’s regulator, Ofgem, awarded a “cap-and-floor” regulatory regime for the Greenlink Interconnector in 2015 (along with other interconnector projects) which will last 25 years. Greenlink is expected to rely on market revenues for most of this period, but the regime provides investor protection in years with low market revenues. In exchange for the revenue floor mechanism, project revenue is also capped.
In other countries and regions, such as India and Texas, mitigating electricity network connection risk has been identified as a priority to drive down both VRE contract prices and reduce flexibility requirements resulting from new VRE installations. India’s Ministry of New and Renewable Energy (MNRE) has introduced a solar park policy which contributes to the target of installing 100 GW of additional solar generation by 2022. Rate payer-funded transmission lines are built to connect these solar parks, providing connection infrastructure for new VRE projects. The policy has attracted investors by removing obstacles to transmission connection, including risks related to permitting and rights of way. Figure 6 shows the weighted average tariffs awarded for projects within different solar parks under the policy between 2015 and 2017, with a trend of declining cost over time. The policy also encourages hybrid projects incorporating wind or storage with PV systems to provide improved flexibility and reduce the variability of VRE generation. As of August 2017, 36 solar parks in 21 Indian states with an aggregate capacity of around 21 GW had been approved and were at various stages of development.
Solar parks have been an effective approach for mitigating grid connection risk and reducing system flexibility requirements in India, while simultaneously driving down VRE procurement costs
Figure 6. Average tariffs awarded to projects under the solar park policy
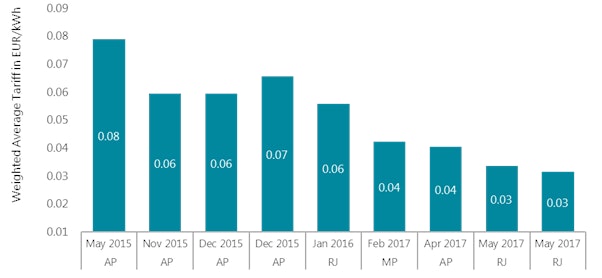
Source: Meena et al. (2017), Success story of solar parks in India. Notes: Each tariff refers to a different solar park within each state; AP = Andhra Pradesh; RJ = Rajasthan; MP = Madhya Pradesh.
Greater inter-regional and international co-ordination can unlock flexibility and yield significant economic benefits
Transmission interconnectors can enable the sharing of flexibility resources across diverse geographies and jurisdictions, including those with distinct market rules and governance structures. In many cases, it is important for policy makers to play a co ordination role to ensure the distinct policy frameworks and institutional arrangements of each power system are sufficiently harmonised to ensure flexibility resources can be effectively shared.
A recent IEA report explored the impact of continuing the practice of limited regional co-ordination in China in 2035, based on the World Energy Outlook’s New Policies Scenario 25. Currently, inter-regional trade is constrained by factors, including limited joint governance over the operation of provincial systems, and the economic interests of individual provinces to use their own generation as opposed to importing from other regions. Modelling results from the study show that removing existing barriers to inter-regional co ordination and power trading in China would result in an annual operational cost savings of USD 3.5 (United States dollars) per megawatt hour (Figure 7; 26), which is equivalent to USD 9 billion. This cost reduction is largely driven by reduced coal consumption, which is replaced by electricity generated from VRE. Annual CO2 emissions are reduced by almost 500 million tonnes due to less coal-fired generation. A recent study by Enel and the Centro Elettrotecnico Sperimentale Italiano (CESI) shows similar results concerning the role of grids to integrate an additional 1.5.GW of VRE capacity in both Chile and Argentina by 2030 27.
A greater degree of inter-regional co ordination and power trading can bring substantial cost savings and emission reductions by sharing flexibility resources more widely.
Figure 7. China’s annual power system operational costs and CO2 emissions, 2035
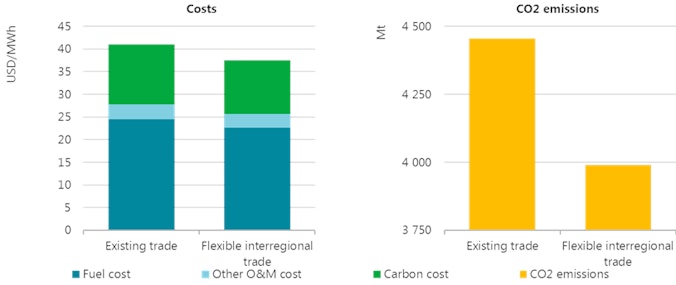
Source: IEA (2019a), China Power System Transformation: Assessing the Benefit of Optimised Operations and Advanced Flexibility
The utilisation of inter-regional energy imbalance mechanisms can also help to mitigate system flexibility requirements and unlock latent flexibility resources. Under these constructs, neighbouring power systems are not fully integrated in their operations per se, but instead share resources that help net out differences between their scheduled electricity production/demand expectations and actual power system requirements. In the United States, the western synchronous interconnection does not have a single organised market, but instead uses a voluntary Energy Imbalance Market that covers participants in eight western states. In Europe, the International Grid Control Cooperation project involves a mechanism for a cross-border imbalance netting process, effectively creating larger balancing areas and promoting system flexibility.
Transmission system operators (TSOs) in different countries can also co-ordinate to improve the development and use of resources across international boundaries. Europe is perhaps the most advanced region for cross-border power system integration. Countries are well interconnected, day-ahead markets are harmonised, and, in some cases, balancing markets are also harmonised across borders. These efforts have led to measurable benefits: the European Network of Transmission System Operators for Electricity (ENTSO-E) estimates that day-ahead market integration has led to approximately EUR 1 billion (Euro) in increased social welfare (due to lower wholesale electricity prices) 28.
4. Battery energy storage systems
Battery energy storage systems are becoming a cost-competitive flexibility provider
While PSH is still the most widely deployed utility-scale storage option, a rapid decline in technology costs is creating an important opportunity for BESS to play a larger role in providing power system flexibility. BESS offer notably fast and accurate response times to dispatch signals from system operators, and their modularity enables a wide range of installation sizes and potential locations for deployment.
Battery costs have declined considerably29 but in most contexts BESS are not yet a fully cost-competitive flexibility resource. While further reducing costs and improving the technology’s performance characteristics remain important, it is equally important to ensure that policy, market and regulatory frameworks allow BESS to participate fairly within the power sector, and offer the full range of services they are technically capable of providing.
Increasing investment in grid-scale batteries in power systems around the world is an important trend and has a significant potential to increase system flexibility. In recent years a number of large grid-scale battery projects have been developed, including in Australia, California, Chile, Illinois, Italy, Puerto Rico and West Virginia.
Changes to connection codes and market rules enable participation by energy storage resources
Changes to established market rules may be required for energy storage resources to be able to participate in the power system. Importantly, such modifications would seek to ensure the eligibility of storage to participate in the power system in a way that recognises the unique technical and operational characteristics of this resource class. What these changes look like in practice, however, depends on the market context.
For settings with wholesale energy markets, regulators and market operators will likely need to collaborate to implement changes to various market rules, including aspects (e.g. bidding parameters) to ensure that storage can act as both a wholesale buyer and seller of electricity. In the United States, for example, the Federal Energy Regulatory Commission (FERC) issued Order 841 in February 2018, which directs independent system operators (ISOs) and regional transmission organisations (RTOs) to open wholesale energy, ancillary service and capacity markets to energy storage resources 30. December 2019 is the deadline to implement these rule changes, which are expected to enable a significant increase in the participation of batteries in electricity markets across the United States.
For vertically-integrated and/or single-buyer markets, centralised utility procurement processes are often the “point of market entry” for many power system resources. Modifications to procurement practices may therefore be the relevant point of intervention for policy makers and regulators to consider.
Regulatory innovation can unlock the multiple benefits of storage resources
It is important to note that one of the key advantages to storage (and particularly battery energy storage) is its technical capability to offer multiple sources of value for the power system. This includes providing energy and ancillary services to the bulk power system, addressing congestion at the distribution and transmission levels, contributing to meeting reserve requirements, and helping to manage energy usage for individual customers. In this context, storage is often discussed as having the ability to “stack value” to provide services and derive revenue from multiple applications. However, in some contexts, policy, market and regulatory frameworks do not allow technically capable resources to provide multiple services simultaneously, and the full economic value of storage will only be realised if these frameworks are modified.
Substantial cost reductions in power system operation can be achieved through allowing batteries and demand-side response to enter markets.
Figure 8. Supply curve for South Australia ancillary services market
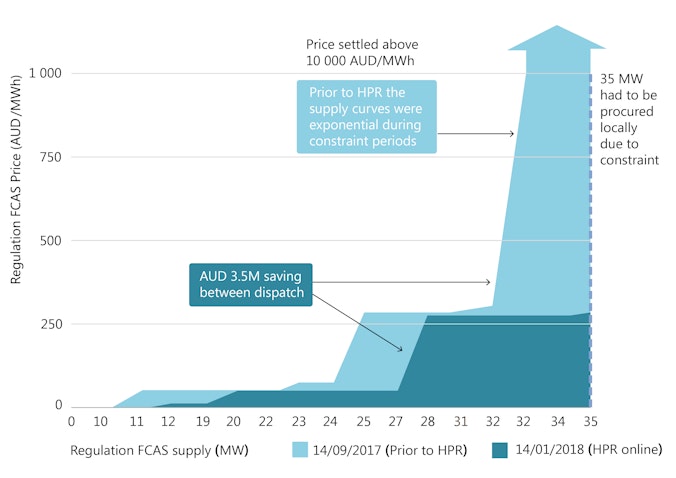
Source: Aurecon (2018), Hornsdale Power Reserve: Year 1 Technical and Market Impact Case Study.
A number of policy or regulatory changes taking place in different parts of the world are enabling benefit-stacking. One notable example is the Hornsdale Power Reserve (HPR)31 in Australia – one of the world’s largest lithium-ion batteries – which participates in regulation, contingency reserve and energy markets in the Australian National Electricity Market (NEM). Built with support from the State Government of South Australia to improve power system security, the battery has multiple value streams, which are made possible through a combination of multiple overlaying contracts attached to particular capacity blocks of the battery. In this case, the State Government has reserved 70 MW for improved system security, including frequency control and participation in the System Integrity Protection Scheme. The remaining 30 MW are contracted by the developer, Neoen, for arbitrage in the energy market and participation in the eight frequency control ancillary services (FCAS) markets. It should be noted that this comprehensive combination of revenue streams was enabled through active co ordination between the State Government, Neoen and AEMO, but the underlying market rules would still allow future battery storage projects to simultaneously participate in the energy and ancillary services markets. HPR’s deployment has successfully contributed to improve system security. The battery also helped to drive down FCAS prices (Figure 8), due to increased competition in the FCAS market during periods where FCAS services had to be provided locally.
In markets with pre-existing barriers for benefit-stacking in battery storage deployment, regulatory changes may be needed before batteries can be used to their full potential. Measures may include removing exclusivity clauses in ancillary services contracts, changing the specifications of flexibility services to allow for participation in multiple services, or redefining the role of storage owners to allow their participation in additional markets. Examples of adaptations to make better use of batteries include the ongoing changes to balancing market access regulations and flexibility product design in the United Kingdom, and the special provisions enabling the ConEd GI project to participate in both regulated and competitive market segments.
Technology and policy innovations can help accelerate the deployment of storage to serve long-term flexibility needs
While batteries have significant potential for providing short-term flexibility services, many power systems need to continue to explore solutions to meet mid-to long-term flexibility needs. Technology options, such as pumped storage hydro (PSH), large reservoir hydropower and “power-to-X” technologies (e.g. electrolytic hydrogen production), may be able to address longer-term flexibility issues, such as seasonal imbalances in VRE production. These options are likely to become more important with increasing VRE shares. However, hydropower technologies are limited to suitable sites, and other longer-term storage options are largely not cost-effective at this stage.
On this basis, there is an important role for providing support for pilots of energy storage technologies with longer storage durations. The Advanced Research Projects Agency – Energy (ARPA-E) in the United States is currently operating a programme called “Duration Addition to electricitY Storage (DAYS)” to develop storage systems with durations of between ten to 100 hours 32. Around half the projects that have successfully obtained funding utilise some form of thermal storage; other projects include, a flow battery, a fuel cell and a type of pumped hydropower resource that depends on pumping pressurised water underground.
The second element, which is crucial to enable the cost-effective deployment of long-term energy storage technologies, is ensuring technology-neutral remuneration of flexibility. Economic challenges for long-term storage assets are perhaps best illustrated by pumped storage hydropower resources. Although deployment rates have declined in recent years, in 2017 PSH was still the largest source of new energy storage and new projects are being planned and built in countries and regions, including Australia, Austria, China, Portugal, Southeast Asia, Switzerland and the United Kingdom 33, 34. However, doubt exists around the current and future profitability of PSH projects, particularly as markets with higher VRE penetrations tend to have reduced financial opportunities for energy arbitrage activities. Policy makers may need to explore methods to provide a market-based remuneration for the longer-term flexibility services offered by PSH and other long-term storage technologies; this could offer additional revenue opportunities for existing facilities, as well as send appropriate investment signals to developers of new projects.
5. Distributed energy resources
Distributed energy resources offer significant flexibility potential but may require market and regulatory reforms
DER, such as distributed generation, distributed battery storage, demand response and electric vehicles, are poised for significant growth in the coming decades. While DER have a number of benefits for individual customers, from the power system perspective, DER also have the potential to be aggregated together and leveraged to provide system flexibility services at the local and bulk power system levels. However, seizing these opportunities may require various changes to connection codes, regulation and market rules. One example of DER deployment is the Fortum Spring virtual battery, which aggregates the loads of electric water heaters in Finland (Figure 9).
Changes to legal frameworks and connection codes enabling aggregation are key for DER resources to provide flexibility services
Figure 9. Locations of distributed hot water systems enrolled in the Fortum Spring virtual battery which provide flexibility to the Finnish power reserve market
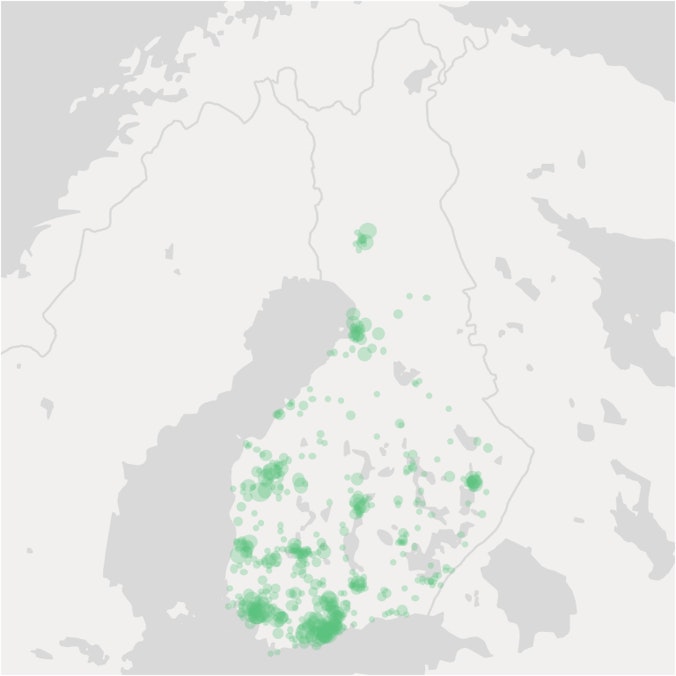
Source: Fortum (2019). Fortum Spring.
Realising DER benefits depends on a variety of market rules and regulations that enable their participation
One common barrier to the enrolment of DER as a flexibility resource is the high transaction costs associated with “qualifying” these small-scale resources for market participation. In the absence of proactive changes to market and/or connection codes, individual DER may often be subject to the same qualification requirements as larger-scale resources. Modifications to the qualification requirements may therefore be necessary. In Ireland, for example, changes under the DS3 programme have enabled the participation of distributed generation resources in the provision of short-term flexibility services. In Germany, the concept of group-based pre-qualification criteria is an emerging topic for the use of electric vehicle EV fleets in flexibility service provision.35This would, for example, facilitate the administrative pre-approval of all charging points of a specific design, rather than the individual review of each charging point for standards compliance.
In settings with competitive wholesale markets, new legal or administrative efforts may be required to allow aggregators to participate in the energy and/or ancillary services market. In Europe, the Clean Energy Package’s Electricity Directive sets out a common definition of an aggregator, along with a set of their rights and obligations 36.
For regulated power market segments, such as distribution or transmission and/or in settings with vertically integrated or single-buyer utilities, the point of market entry for DER are often utility-driven planning and procurement processes. In this context, it may be necessary to examine the incentives that regulated utilities have to consider DERs as a source of flexibility in system planning as an alternative to traditional investments in the power system. Utilities typically earn a fixed return on their approved capital expenditures (CAPEX), based on their role in building and operating their own infrastructure. The deployment of aggregated DER to provide localised flexibility services, however, would generally be classified as an operational expenditure (OPEX), which is typically not considered as part of the revenues’ utilities are allowed to receive. This may lead utilities to prioritise traditional network investments, even in scenarios where the procurement of flexibility services from DER could help to defer or avoid investments in networks. In this case, specific regulatory measures may be introduced to re-orient utility incentives. One approach to addressing this issue is to remove the distinction between CAPEX and OPEX when examining utilities. The UK regulator, Ofgem, has mandated a transition toward a “total expenditure framework”, or “TOTEX”, which grants utilities a single expenditure allowance for maintaining network infrastructure. Another approach is for policy makers to mandate consideration of innovative DER solutions during utility planning exercises as an alternative to traditional network investment, as is happening increasingly in Australia, the United States and elsewhere. For example, New York’s Public Service Commission has introduced a shared savings provision to encourage the deployment of so-called “non-wire alternatives”, requiring distribution utilities to consider DER solutions as alternatives to traditional network upgrades.
Testing innovative approaches can help broaden understanding of specific opportunities for DER deployment for system flexibility
Global experience suggests that the specific barriers to enabling DER aggregation vary significantly across power sector jurisdictions. Thus, studying and/or piloting particular approaches to DER aggregation may be a valuable strategy for policy makers to identify opportunities.
For instance, for the Lombardy region of Italy, the publicly funded research agency RSE conducted a feasibility study for a Virtual Storage System which would comprise more than 500 “behind-the-meter” BESS and solar PV systems. Specifically, the study characterised the flexibility potential, business case and associated regulatory barriers to aggregating the systems and allowing them to bid flexibility services into the Italian Ancillary Services Market. The results of the study indicated that it would be possible to make a strong business case for a prospective virtual storage system, even in light of the additional costs of the measurement and control systems that would be required to enable aggregation. It was also found that under the current retail tariff structure, customers with behind-the-meter BESS and solar PV systems would be disincentivised to provide flexibility services, and that new regulations must be developed to address this disincentive.
Another example of testing innovative approaches can be observed in Germany, where the SINTEG project has enabled a number of so-called “regulatory sandboxes” to test the feasibility of new flexibility solutions in specific regions. One such project in north western Germany, known as the Enera project, is being tested in a location where local VRE generation is equivalent to 235% of the local yearly demand. The project aims to avoid short-term distribution network congestion and redispatch costs by piloting a local flexibility services platform for market-based congestion management. EpexSpot, the platform operator, acts as a neutral intermediary between local transmission and distribution network operators (Tennet, Avacon, and EWE Netz), who would otherwise be liable for redispatch costs and local flexibility providers, who are expected to deliver a more cost-efficient alternative. The platform relies on two conditions for an efficient flexibility market: network congestion and competition. The first condition relates to the need for a remedial measure outside of normal operation conditions, while the second requires enough flexibility providers so that the dispatch of local flexibility is indeed least-cost.
Following a two-year conception phase, the project went live in February 2019, carrying out the first flexibility trade through the activation of a local power-to-gas facility. With a rising number of participants and flexibility transactions, the project aims to highlight the economic benefit of market-based flexibility as an alternative to traditional network upgrades or wind plant curtailment.
Sector coupling efforts have the potential to enrol new flexible loads at scale to enhance power system flexibility
“Sector coupling” has been identified as a key strategy to promote economy-wide decarbonisation and broader macro-economic efficiency. It is defined as the intelligent linkage between the power sector and other energy-consuming sectors (e.g. industry, mobility and buildings), often through advanced sensing, communication and control technologies, that flexibly utilises demand to integrate VRE and lower power system operational costs. Sector coupling offers a significantly increased potential to reduce primary energy demand (through efficiencies and fuel switching) and “flexibilise” the demand-side of the power system flexibility, while also supporting power sector revenue sufficiency through electrification efforts which increase demand (Figure 10). A new Clean Energy Ministerial horizontal accelerator focused on sector coupling will be considered in 2019 to broaden understanding and share experiences of this trend.
Sector coupling offers a significant potential to “flexibilise” the demand-side of the power system and promote economy-wide decarbonisation.
Figure 10. Conceptual diagram of sector coupling
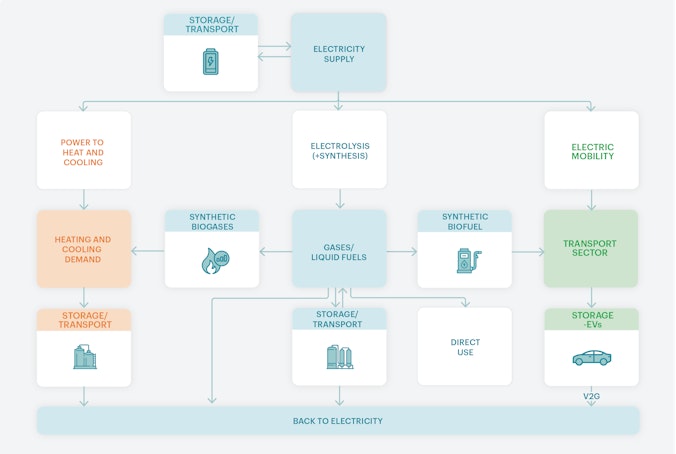
IEA. All rights reserved.
References
IEA (International Energy Agency) and 21CPP (2018), Status of Power System Transformation 2018 – Advanced Power Plant Flexibility, , https://webstore.iea.org/status-of-power-system-transformation-2018.
IEA (2019a), China Power System Transformation – Assessing the Benefit of Optimised Operations and Advanced Flexibility Options, , https://webstore.iea.org/china-power-system-transformation.
For further details, see IEA and 21CPP (2018), Status of Power System Transformation 2018 – Advanced Power Plant Flexibility, , https://webstore.iea.org/status-of-power-system-transformation-2018.
IEA (2018b), World Energy Investment 2018, https://webstore.iea.org/world-energy-investment-2018.
21CPP (2015), Status Report on Power System Transformation: A 21st Century Power Partnership Report, NREL, Golden, CO, www.nrel.gov/docs/fy15osti/63366.pdf.
IEA and 21CPP (2018), Status of Power System Transformation 2018 – Advanced Power Plant Flexibility, https://webstore.iea.org/status-of-power-system-transformation-2018.
IEA and 21CPP (2017), Status of Power System Transformation 2017 – System Integration and Local Grids, https://webstore.iea.org/status-ofpower-system-transformation-2017.
EMA (Energy Market Authority) (2018), Electricity Liberalisation, www.ema.gov.sg/electricity_market_liberalisation.aspx.
Importantly, this is only a relevant point of intervention for jurisdictions with competitive markets.
Wholesale markets in the United States are operated by independent system operators (ISOs), which include ancillary services, whereas in European markets, wholesale energy markets are typically operated by power exchanges whilst ancillary service markets are typically procured by transmission system operators (TSOs).
Elexon (2018), Imbalance Pricing, www.elexon.co.uk/operations-settlement/balancing-and-settlement/imbalance-pricing.
AEMO (Australian Energy Market Operator) (2018), Integrated System Plan July 2018: For the National Electricity Market, Australia Energy Market Operator, www.aemo.com.au/-/media/Files/Electricity/NEM/Planning_and_Forecasting/ISP/2018/Integrated-System-Plan-2018_final.pdf.
UNU-WIDER (United Nations University World Institute for Development Economics Research) (2017), The Political Economy of Clean Energy Transitions, the United Nations University World Institute for Development Economics Research, Oxford University Press.
ARPA-E (Advanced Research Projects Agency-Energy) (2018), DAYS – Duration Addition to Electricity Storage, , https://arpa-e.energy.gov/?q=arpa-e-programs/days.
IEA (2017), Getting Wind and Sun onto the Grid: A Manual for Policy Makers, , www.iea.org/publications/insights/insightpublications/Getting_Wind_and_Sun.pdf.
IRENA (International Renewable Energy Agency) (2016), Scaling up Variable Renewable Rower: The Role of Grid Codes, IRENA, www.irena.org/-/media/Files/IRENA/Agency/Publication/2016/IRENA_Grid_Codes_2016.pdf.
Energy storage systems co-located with VRE resources can ease the offering of more advanced ancillary services.
CAISO (California Independent System Operator) (2017), News Release: Tests Show Renewable Plants Can Balance Low-Carbon Grid, California Independent System Operator, www.caiso.com/documents/testsshowrenewableplantscanbalancelow-carbongrid.pdf.
Gevorgian, V., O’Neill, B. (2016), Advanced Grid-Friendly Controls Demonstration Project for Utility-Scale PV Power Plants, , www.nrel.gov/docs/fy16osti/65368.pdf.
Energy and Environmental Economics, Inc. (2018), Investigating the Economic Value of Flexible Solar Power Plant Operation, Energy and Environmental Economics, San Francisco, www.ethree.com/wp-content/uploads/2018/10/Investigating-the-Economic-Value-of-Flexible-Solar-Power-Plant-Operation.pdf.
Enel Foundation and HTERI (Huaneng Technical Economics Research Institute) (2019), Power Market Designs for Energy Transition in the EU and China, , www.enelfoundation.org/content/dam/enel-found/EF_PowerMarkets_web.pdf.
IEA (2014), The Power of Transformation – Wind, Sun and the Economics of Flexible Power Systems, , https://webstore.iea.org/the-power-of-transformation.
IEA (2016), Next Generation Wind and Solar Power – From Cost to Value, , www.iea.org/publications/freepublications/publication/Next_Generation_Windand_Solar_PowerFrom_Cost_to_ValueFull_Report.pdf.
More information is available at: www.nationalgrid.com/document/118641/download.
Greenlink (2018), TEN-E Regulation Information Brochure, Issue 2, November 2018, , www.greenlink.ie/sites/default/files/downloads/Greenlink%20Interconnector%20Brochure%20-%20Issue%202%20-%20Website.pdf.
IEA (2019a), China Power System Transformation – Assessing the Benefit of Optimised Operations and Advanced Flexibility Options, , https://webstore.iea.org/china-power-system-transformation.
IEA (2019a), China Power System Transformation – Assessing the Benefit of Optimised Operations and Advanced Flexibility Options, , https://webstore.iea.org/china-power-system-transformation.
Enel Foundation and CESI (Centro Elettrotecnico Sperimentale Italiano) (2019), "Variable Renewable Energy Sources (VRES) deployment and role of interconnections lines for their optimal exploitation – the Chile-Argentina case study", , www.enelfoundation.org/topic/a/2019/05/new-series-of-studies-on-potential-for-renewable-development-and.
ENTSO-E (European Network of Transmission System Operators for Electricity) (2017), Time to Team Up and Create Value, Network Codes Conference, Brussels, https://docstore.entsoe.eu/Documents/Network%20codes%20documents/General%20NC%20doc uments/20170505%20Network%20Codes%20Conference.pdf.
A recent report by Bloomberg New Energy Finance suggests that the levelised cost of electricity from lithium-ion battery storage has dropped by 76% since 2012. See: https://about.bnef.com/blog/battery-powers-latest-plunge-costs-threatens-coal-gas.
FERC (Federal Energy Regulatory Commission) (2016), Order Accepting Proposed Tariff Revisions Subject to Condition, FERC, www.ferc.gov/CalendarFiles/20160602164336-ER16-1085-000.pdf .
The battery is rated at 100 MW discharge capacity, 80 MW charging capacity with 129 MWh storage.
ARPA-E (Advanced Research Projects Agency-Energy) (2018), DAYS – Duration Addition to Electricity Storage, , https://arpa-e.energy.gov/?q=arpa-e-programs/days.
IEA (2018b), Renewables 2018 – Analysis and forecasts to 2023, , www.iea.org/renewables2018 .
IEA (2018c), World Energy Investment 2018, , https://webstore.iea.org/world-energy-investment-2018.
Global EV Outlook 2019 (IEA, 2019b) examines a number of relevant flexibility and system integration issues and opportunities related to EV deployment.
Meus, L. and Nouicer, A. (2018), The EU Clean Energy Package, , http://cadmus.eui.eu/bitstream/handle/1814/57264/RSCAS_2018_TechnicalReport.pdf?sequence=1&isAllowed=y.
Figures sources:
Full references for the data behind IEA figures and/or tables featured on this page can be found in the PDF of the full report.
Reference 1
IEA (International Energy Agency) and 21CPP (2018), Status of Power System Transformation 2018 – Advanced Power Plant Flexibility, , https://webstore.iea.org/status-of-power-system-transformation-2018.
Reference 2
IEA (2019a), China Power System Transformation – Assessing the Benefit of Optimised Operations and Advanced Flexibility Options, , https://webstore.iea.org/china-power-system-transformation.
Reference 3
For further details, see IEA and 21CPP (2018), Status of Power System Transformation 2018 – Advanced Power Plant Flexibility, , https://webstore.iea.org/status-of-power-system-transformation-2018.
Reference 4
IEA (2018b), World Energy Investment 2018, https://webstore.iea.org/world-energy-investment-2018.
Reference 5
21CPP (2015), Status Report on Power System Transformation: A 21st Century Power Partnership Report, NREL, Golden, CO, www.nrel.gov/docs/fy15osti/63366.pdf.
Reference 6
IEA and 21CPP (2018), Status of Power System Transformation 2018 – Advanced Power Plant Flexibility, https://webstore.iea.org/status-of-power-system-transformation-2018.
IEA and 21CPP (2017), Status of Power System Transformation 2017 – System Integration and Local Grids, https://webstore.iea.org/status-ofpower-system-transformation-2017.
Reference 7
EMA (Energy Market Authority) (2018), Electricity Liberalisation, www.ema.gov.sg/electricity_market_liberalisation.aspx.
Reference 8
Importantly, this is only a relevant point of intervention for jurisdictions with competitive markets.
Reference 9
Wholesale markets in the United States are operated by independent system operators (ISOs), which include ancillary services, whereas in European markets, wholesale energy markets are typically operated by power exchanges whilst ancillary service markets are typically procured by transmission system operators (TSOs).
Reference 10
Elexon (2018), Imbalance Pricing, www.elexon.co.uk/operations-settlement/balancing-and-settlement/imbalance-pricing.
Reference 11
AEMO (Australian Energy Market Operator) (2018), Integrated System Plan July 2018: For the National Electricity Market, Australia Energy Market Operator, www.aemo.com.au/-/media/Files/Electricity/NEM/Planning_and_Forecasting/ISP/2018/Integrated-System-Plan-2018_final.pdf.
Reference 12
UNU-WIDER (United Nations University World Institute for Development Economics Research) (2017), The Political Economy of Clean Energy Transitions, the United Nations University World Institute for Development Economics Research, Oxford University Press.
Reference 13
ARPA-E (Advanced Research Projects Agency-Energy) (2018), DAYS – Duration Addition to Electricity Storage, , https://arpa-e.energy.gov/?q=arpa-e-programs/days.
Reference 14
IEA (2017), Getting Wind and Sun onto the Grid: A Manual for Policy Makers, , www.iea.org/publications/insights/insightpublications/Getting_Wind_and_Sun.pdf.
Reference 15
IRENA (International Renewable Energy Agency) (2016), Scaling up Variable Renewable Rower: The Role of Grid Codes, IRENA, www.irena.org/-/media/Files/IRENA/Agency/Publication/2016/IRENA_Grid_Codes_2016.pdf.
Reference 16
Energy storage systems co-located with VRE resources can ease the offering of more advanced ancillary services.
Reference 17
CAISO (California Independent System Operator) (2017), News Release: Tests Show Renewable Plants Can Balance Low-Carbon Grid, California Independent System Operator, www.caiso.com/documents/testsshowrenewableplantscanbalancelow-carbongrid.pdf.
Reference 18
Gevorgian, V., O’Neill, B. (2016), Advanced Grid-Friendly Controls Demonstration Project for Utility-Scale PV Power Plants, , www.nrel.gov/docs/fy16osti/65368.pdf.
Reference 19
Energy and Environmental Economics, Inc. (2018), Investigating the Economic Value of Flexible Solar Power Plant Operation, Energy and Environmental Economics, San Francisco, www.ethree.com/wp-content/uploads/2018/10/Investigating-the-Economic-Value-of-Flexible-Solar-Power-Plant-Operation.pdf.
Reference 20
Enel Foundation and HTERI (Huaneng Technical Economics Research Institute) (2019), Power Market Designs for Energy Transition in the EU and China, , www.enelfoundation.org/content/dam/enel-found/EF_PowerMarkets_web.pdf.
Reference 21
IEA (2014), The Power of Transformation – Wind, Sun and the Economics of Flexible Power Systems, , https://webstore.iea.org/the-power-of-transformation.
Reference 22
IEA (2016), Next Generation Wind and Solar Power – From Cost to Value, , www.iea.org/publications/freepublications/publication/Next_Generation_Windand_Solar_PowerFrom_Cost_to_ValueFull_Report.pdf.
Reference 23
More information is available at: www.nationalgrid.com/document/118641/download.
Reference 24
Greenlink (2018), TEN-E Regulation Information Brochure, Issue 2, November 2018, , www.greenlink.ie/sites/default/files/downloads/Greenlink%20Interconnector%20Brochure%20-%20Issue%202%20-%20Website.pdf.
Reference 25
IEA (2019a), China Power System Transformation – Assessing the Benefit of Optimised Operations and Advanced Flexibility Options, , https://webstore.iea.org/china-power-system-transformation.
Reference 26
IEA (2019a), China Power System Transformation – Assessing the Benefit of Optimised Operations and Advanced Flexibility Options, , https://webstore.iea.org/china-power-system-transformation.
Reference 27
Enel Foundation and CESI (Centro Elettrotecnico Sperimentale Italiano) (2019), "Variable Renewable Energy Sources (VRES) deployment and role of interconnections lines for their optimal exploitation – the Chile-Argentina case study", , www.enelfoundation.org/topic/a/2019/05/new-series-of-studies-on-potential-for-renewable-development-and.
Reference 28
ENTSO-E (European Network of Transmission System Operators for Electricity) (2017), Time to Team Up and Create Value, Network Codes Conference, Brussels, https://docstore.entsoe.eu/Documents/Network%20codes%20documents/General%20NC%20doc uments/20170505%20Network%20Codes%20Conference.pdf.
Reference 29
A recent report by Bloomberg New Energy Finance suggests that the levelised cost of electricity from lithium-ion battery storage has dropped by 76% since 2012. See: https://about.bnef.com/blog/battery-powers-latest-plunge-costs-threatens-coal-gas.
Reference 30
FERC (Federal Energy Regulatory Commission) (2016), Order Accepting Proposed Tariff Revisions Subject to Condition, FERC, www.ferc.gov/CalendarFiles/20160602164336-ER16-1085-000.pdf .
Reference 31
The battery is rated at 100 MW discharge capacity, 80 MW charging capacity with 129 MWh storage.
Reference 32
ARPA-E (Advanced Research Projects Agency-Energy) (2018), DAYS – Duration Addition to Electricity Storage, , https://arpa-e.energy.gov/?q=arpa-e-programs/days.
Reference 33
IEA (2018b), Renewables 2018 – Analysis and forecasts to 2023, , www.iea.org/renewables2018 .
Reference 34
IEA (2018c), World Energy Investment 2018, , https://webstore.iea.org/world-energy-investment-2018.
Reference 35
Global EV Outlook 2019 (IEA, 2019b) examines a number of relevant flexibility and system integration issues and opportunities related to EV deployment.
Reference 36
Meus, L. and Nouicer, A. (2018), The EU Clean Energy Package, , http://cadmus.eui.eu/bitstream/handle/1814/57264/RSCAS_2018_TechnicalReport.pdf?sequence=1&isAllowed=y.
Reference 37
Reference 38
Figures sources:
Full references for the data behind IEA figures and/or tables featured on this page can be found in the PDF of the full report.