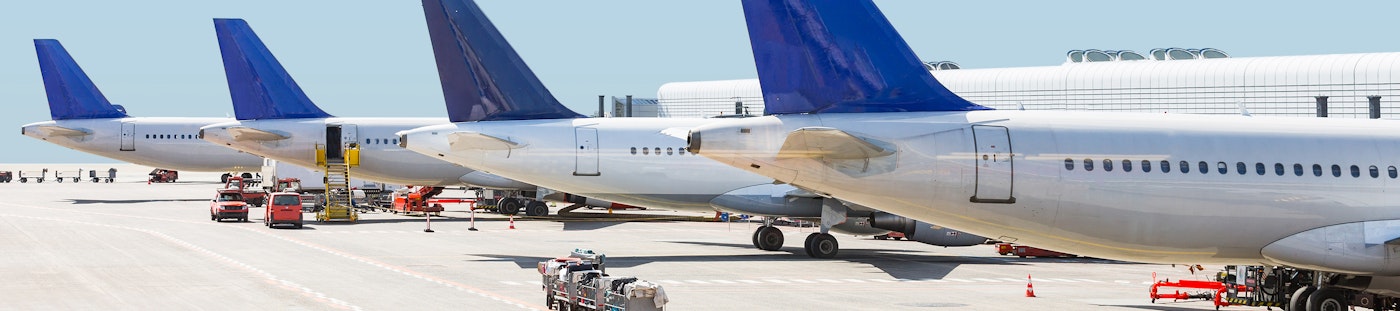
Aviation
Why is aviation important?
Aviation accounts for a relatively small share of global emissions but is one of the most challenging sectors to decarbonise. Despite reductions in flying during the Covid-19 lockdowns, demand is expected to grow rapidly through 2030. New aircraft can be up to 20% more efficient than the models they replace, but growth in activity has historically outpaced efficiency improvement.
Where do we need to go?
Technology innovation is needed across the sector, including in production of low-emission fuels, improvements in aircraft and engines, and operational optimisation. Demand restraint solutions will also be needed to get on track with the Net Zero Emissions by 2050 Scenario – to curb growth in emissions and ultimately reduce them this decade.
What are the challenges?
Planned production capacity for sustainable aviation fuels will provide just a small fraction of jet fuel demand by 2027. Increasing the use of these fuels to get in line with the Net Zero Scenario will require supportive policies and a significant ramp-up of investments in production capacity.
Tracking Aviation
In 2022 aviation accounted for 2% of global energy-related CO2 emissions, having grown faster in recent decades than rail, road or shipping. As international travel demand recovers following the Covid-19 pandemic, aviation emissions in 2022 reached almost 800 Mt CO2, about 80% of the pre-pandemic level. Many technical measures related to low-emission fuels, improvements in airframes and engines, operational optimisation and demand restraint solutions are needed to curb growth in emissions and ultimately reduce them this decade in order to get on track with the Net Zero Emissions by 2050 (NZE) Scenario.
Political agreement on targeting net zero for aviation has been complemented by fiscal and regulatory policies to promote sustainable aviation fuels in major markets
Notable progress towards getting aviation on track includes the following:
- The 184 member states of the International Civil Aviation Organization (ICAO) adopted in 2022 a long-term global aspirational goal (LTAG) of net zero carbon emissions from international aviation by 2050.
- In 2022 the United States announced important tax credits and a competitive grant programme under the Inflation Reduction Act, which will allocate USD 3.3 billion to scaling up sustainable aviation fuel (SAF) production, with the aim of meeting its 3 billion gallons milestone by 2030.
- In the European Union, the European Parliament and Council reached a political agreement in 2023 on the rules of ReFuelEU Aviation on the schedule of minimum SAF blend-in shares, with sub-targets for synthetic fuels, through 2050.
- In 2022, following its announced Jet Zero pledge, the United Kingdom dedicated GBP 165 million to support SAF projects, with a plan to have at least 5 commercial SAF plants under construction by 2025.
CO2 emissions from aviation have reached 80% of their pre-pandemic peak
CO2 emissions in aviation in the Net Zero Scenario, 2000-2030
OpenAviation emissions rose in 2022 to reach nearly 80% of their pre-pandemic peak in 2019. After increasing at an average of 2.3% per year from 1990 to 2019, direct CO2 emissions from fossil fuel combustion plummeted from more than 1 000 Mt CO2 in 2019 to less than 600 Mt CO2 in 2020 in the context of the Covid-19 pandemic. As demand from air passengers recovered in 2022, emissions increased in all regions with the exception of China (due to the zero-Covid policy) and Russia (due to the invasion of Ukraine), reaching almost 800 Mt CO2. CO2 emissions are expected to grow rapidly and to surpass their 2019 level around 2025.
Multiple measures are required to promote the technologies, sustainable aviation fuels (SAF) and demand-side management needed to bring the currently rising emissions level below 1 000 Mt CO2 by 2030, in line with the NZE Scenario. Policy and fiscal support should prioritise improvements in energy efficiency, stimulating investment in pre-commercial and low-emissions SAF, and developing alternatives to jet kerosene, such as electricity- or hydrogen-powered aircraft.
Improvements in energy intensity have not been sufficient to counterbalance energy demand growth in recent years
Energy intensity of commercial passenger aviation in the Net Zero Scenario, 2000-2030
OpenFrom 2010 to 2019, average fuel efficiency per revenue tonne kilometre (RTK) equivalent travelled improved by 1.8% per year thanks to the introduction of more efficient aircraft and engines, with improvements over the decade nearly reaching ICAO’s aspirational goal of 2% per annum through 2050. However, efficiency improvements have not kept up with demand growth, which grew at an average rate of over 5% annually between 2010 and 2019. To stay on track with the Net Zero Scenario, efficiency will need to improve at a rate of 2% per year through 2030, on a par with the historical average improvements over the past two decades.
In addition to technical efficiency improvements in engine and airframe designs, improvements in payload and traffic efficiency (i.e. the weight of cargo and number of passengers carried per aircraft) have also contributed to reducing the energy intensity of aircraft operation. Payload efficiency deteriorated between 2020 and 2022, as planes were flown with fewer passengers despite some compensatory increase in freight payloads in the bellies of passenger aircraft.
International passenger activity more than doubled in 2022 compared to the previous year, closing the gap with pre-Covid levels
Global total commercial air passenger traffic, 2019-2022
OpenTotal commercial (international plus domestic) air passenger activity increased by around 70% in 2022, and now stands at three-quarters of pre-pandemic levels. Increasing activity in international aviation was the main driver, with more than 150% growth, after a year of slower recovery compared to domestic aviation. The reopening of international travel in China provides an outlook for even higher growth from the beginning of 2023, as passenger and cargo activity return to pre-pandemic levels.
Air cargo contracted by 8% in 2022 after a historical peak in 2021, as a result of high inflation, disruption of trade flows due to the war in Ukraine, and the strong US dollar making commodities traded in US currency more expensive.
Sustainable aviation fuels are critical to decarbonising aviation
Currently, demand for aviation fuel is dominated by jet kerosene, while SAF account for less than 0.1% of all aviation fuels consumed. Manufacturers and operators are increasingly testing flights that are entirely fuelled by SAF, which can be deployed in current infrastructure, engines and aircraft with minor adjustments to fuel delivery equipment. However, planned production capacities will provide just 1-2% of jet fuel demand by 2027. Increasing SAF use in aviation to 10% by 2030 in line with the Net Zero Scenario will require a significant ramp-up of investment in capacity to produce SAF and supportive policies such as fuel taxes and low-carbon fuels standards.
Commercial adoption of SAF will be driven by policies such as the aforementioned incentives for SAF production in the United States and the United Kingdom, and the European Union’s proposed ReFuelEU regulation. The current ReFuelEU Aviation proposal excludes crop-based SAF due to sustainability concerns, and the potential to scale up biojet kerosene supply based on animal fat and waste oil feedstocks (which are included in the proposed regulation) is limited by their supply. Supporting research and development on advanced biofuel technologies can unlock more abundant and cheaper feedstocks such as agricultural residues, dedicated energy crops and municipal solid waste. In the longer term, synthetic fuels based on hydrogen produced using electrolysers (and running on low-emissions or renewable electricity), combined with CO2 from biogenic, concentrated waste streams or atmospheric sources can provide an alternative, although commercialisation may be challenging.
Revolutionary designs are needed to achieve efficiency improvements of more than 2% annually in the longer term
Incremental improvements to engines, materials, aerodynamics and mild hybridisation can and should be implemented. However, “revolutionary” designs, such as new airframe configurations to enable further efficiency improvement, and alternative propulsion technologies including electric or hydrogen-powered aircraft, may also play a role.
Tests and prototypes showcase innovations in hydrogen-powered aircraft
Hydrogen can be used via direct combustion in jet engines, or in fuel cells to generate electricity for electric motors, or a combination of the two. However, using hydrogen in aircraft poses significant challenges, including the need for innovative fuel storage and delivery methods, low-cost and lightweight cryogenic tanks, and redesigned airframes to accommodate them. By 2040 hydrogen aircraft are likely to have a maximum range of under 3 500 km, serving at a maximum about half of all fuel consumption in current commercial aviation operations.
Test flights for aircraft equipped with fuel cell-powered electric motors have taken place in early 2023, with ZeroAvia flying a 19-seat aircraft equipped with a hydrogen-electric engine on its left wing, and Universal Hydrogen flying a 40-seat regional aircraft at high altitude, with one of its engines powered by fuel cell. This followed its demonstration of prototype modular capsules that can store liquid hydrogen for up to 100 hours and can be transported by semi-truck.
Demonstrations of alternative propulsion systems have also progressed from late 2022 onwards. Airbus's ZEROe programme produced a prototype of a cryogenic hydrogen tank that allows hydrogen to be carried in its liquid form at extremely low temperatures. Rolls-Royce and easyJet tested combusting hydrogen to run a regional jet engine with hydrogen produced from wind and tidal power. Avio Aero has launched a demonstration programme for megawatt-level hybrid electric propulsion technologies, coupling a propulsion engine with a fuel cell-powered electric motor. H2FLY has begun the integration of a liquid hydrogen storage system tank in its four-seat aircraft with hydrogen-electric propulsion.
Battery electric propulsion is currently limited to very small aircraft and short range
Battery electric aircraft have no direct emissions, potentially much lower operational and maintenance costs (dependent on battery durability), high efficiency and create far lower noise pollution. However, current battery energy density and weight severely restrict the range of battery electric flights and the size of the aircraft. In September 2022 Eviation performed a test flight of its 9-seat electric aircraft, with maximum flight range of around 450 km.
The success of deploying electric aircraft technologies will largely depend on the evolution of battery technologies. The energy density of today’s Li-ion batteries is around 200 Wh/kg (with a current practical limit of 300-400 Wh/kg) at the pack level, but for short-haul flights over 1 000 km, a battery pack energy density of at least 800 Wh/kg would be needed.
World’s first airport liquid hydrogen refuelling facility under construction
Airbus and ArianeGroup are working towards building the first liquid hydrogen refuelling facility for ZEROe aircraft at Blagnac airport in Toulouse, France.
Governments are increasing fiscal support for SAF production and mandating SAF use
Production of SAF gained substantial support through the passage in 2022 of the Inflation Reduction Act (IRA) in the United States, with USD 3.3 billion in tax credits and a competitive grant programme allocated to SAF. The IRA provides USD 1.25 per gallon (USD 0.33 per litre) of SAF produced, provided that the lifecycle emissions are no more than 50% of those of fossil jet kerosene. A supplemental 1 cent credit is available for each percent that the lifecycle emissions reduction exceeds 50%.
In addition to amending the European Union Emissions Trading System to phase out allowances given to the aviation industry by 2026, in Q1 2023 the European Council and Parliament agreed on rules mandating a minimum share of SAF with sub-targets for synthetic fuels. Individual countries such as France and Norway already have SAF blending mandates in place, while Sweden’s GHG emissions intensity reduction target will also drive SAF adoption. In 2023 the Swedish government also announced its aim to invest SEK 15 million (Swedish kronor) annually to support research and development of electric aircraft. The United Kingdom, following its 2022 Jet Zero pledge, has dedicated GBP 165 million to support SAF projects, with funding allocation running to 2025.
In the Asia Pacific region, in 2022 Japan proposed legislation mandating that SAF must account for 10% of aviation fuel by 2030. In the same period the Civil Aviation Administration of China also set ambitions to increase SAF use and lower GHG emissions intensity.
View all aviation policies
International aviation has pledged to reach net zero emissions by 2050
In late 2022, ICAO member states adopted a long-term global aspirational goal (LTAG) to achieve net zero carbon emissions from international aviation by 2050. The agreement aims to reduce emissions within the sector itself (i.e. directly from aviation activity, as opposed to via offsetting emissions through purchase of credits). Although it remains non-binding and lacks intermediate goals, member state governments are expected to produce action plans within their own national timeframe and capabilities.
Additionally, countries agreed on a new baseline for the Carbon Offsetting and Reduction Scheme for International Aviation (CORSIA) at 85% of the 2019 emissions level of international aviation. Emissions beyond this would need to be reduced by using sustainable aviation fuels or by purchasing certified emissions reductions to offset CO2 emissions. ICAO approved addition programmes for CORSIA compliance during the pilot phase, many of which rely on offsets outside the energy sector.
Airlines are moving towards offtake agreements with fuel suppliers to supply SAF
Increasing announcements of SAF offtake agreements between fuel suppliers and airlines marked a stark increase in contracted volume from 9 billion litres in 2021 to 22 billion litres in 2022. The largest offtake volume per year purchase to date was agreed between Gevo and OneWorld Alliance, to be sourced from ethanol from inedible corn products. Many of the contracted volumes have planned delivery after 2025, as new SAF plants take around three years to build after a final investment decision has been taken.
We would like to thank the following external reviewers:
- Lynnette Dray, University College London
- Haldane Dodd, Air Transport Action Group
Recommendations
-
Policies to support SAF consumption and boost growth are needed to make SAF more widely available and economically viable.
On the demand side, blending mandates provide clear long-term demand signals beyond offtake agreements. Low-carbon fuel standards provide less clarity on required volumes, but this is balanced by providing a clear regulatory framework to reduce the lifecycle emissions of SAF. By combining blending mandates with GHG emissions and sustainability criteria, regulatory frameworks such as the proposed ReFuelEU Aviation aim to achieve market certainty at the same time as incentivising uptake of less mature and higher cost SAF production pathways in the mid- to long term.
Funding and financial de-risking will be needed to promote continued innovation around sustainable production processes including novel feedstocks (wastes, residues, marginal land, double cropping) and support the leap from demonstration to commercial plants. This will also be needed to drive investment at all stages of research, and to enable power-to-liquids jet kerosene to scale up rapidly.
Both supply and demand of SAF are highly concentrated in advanced economies today. Meeting the growing demand for air travel in emerging and developing economies will require technical assistance and capacity building to accelerate the availability and use of SAF. Such support can help emerging market and developing economies to leverage domestic natural resource endowments such as biomass, solar and wind, and thereby scale up high value-add industries in the clean energy economy.
-
Taxing GHG emissions beyond the CORSIA scheme is critical to more equitably reflect the climate impacts of air travel. As the additional costs of these taxes are passed on to passengers, they can help curb demand growth, while revenues generated could be used to foster low-emission innovation in SAF production or engine and airframe design.
Since frequent fliers likely account for the majority of commercial aviation emissions, progressive tax rates that increase with flight frequency as well as higher taxes on premium class tickets could discourage excessive flying, especially as jet kerosene is currently taxed at lower levels than residential electricity or automative fuels in many regions. However, changing tax structure is complicated by taxation conditions on international flights agreed in the Chicago Convention of 1944.
-
Certain airlines propose offset options to consumers, such as paying extra towards SAF. Some have set corporate targets to supply increasing shares of fuels with SAF, and are actively seeking offtake agreements that secure demand for nascent SAF markets.
Actions from leading airlines and airports that serve as key international and domestic hubs can generate the market pull that is needed to catalyse adoption of efficient operations, best-in-class technologies and SAF. Those that act early will benefit not only from asserting their leadership in corporate social responsibility, but from being the first to gain experience of innovative practices and technologies that will eventually need to be adopted more widely. Investors, lenders and consumers will be instrumental in driving uptake of SAF by funding airlines and setting corporate travel and freight transport policies.
Programmes and partnerships
Renewables 2022
Biojet fuel demand expands to 3 900 MLPY in our main-case forecast – 37 times the 2021 level – to account for nearly 1% of total jet fuel consumption.
Authors and contributors
Lead authors
Hyeji Kim
Jacob Teter